ANATOMY OVERVIEW
CREDIT
The meninges (singular: meninx) are three membranes covering the brain and spinal cord. From the most outer layer, to the most inner layer, they are the dura mater, arachnoid mater and pia mater.
IMAGE CREDIT
IMAGE CREDIT
A 3D MODEL
The ventricular system consists of four interconnected CSF-containing cavities in the brain:
> Two lateral ventricles (located in the the cerebral hemispheres)
> A single midline third ventricle (near the thalamus)
> A fourth ventricle (near the pons and medulla)
CSF is produced by choroidal plexuses located in the lateral, third and fourth ventricles.
The CSF exits the ventricular system, and enters the subarachnoid circulation via the Foramen of Luksha and Foramen of Magendie (lateral and medial respectively) in the fourth ventricle.
The interventricular foramina (or foramina of Monro, FM) are channels that connect the paired lateral ventricles with the third ventricle at the midline of the brain. This is the level that EVD’s are levelled to and correlated externally with the external auditory meatus
CSF plays a number of homeostatic roles.
Interestingly, by filling the subarachnoid space around the brain and spinal cord, CSF creates buoyancy needed to prevent the weight of the brain from crushing nerve roots and blood vessels against the skull.
The brain weighs ~1400 g in the air, but only effectively about 45 g due to the buoyancy of CSF.
CSF is drained back into the venous system via arachnoid granulations penetrating into the dural venous sinuses.
The blood supply to the brain can be divided into an anterior and posterior circulation.
The anterior circulation is suppled by the internal carotid arteries (ICA). Branching off the ICA on the left and right, forming the anterior circulation, are the anterior cerebral artery (ACA), and the middle cerebral artery (MCA).
Arising from the subclavian arteries, the vertebral arteries (VA) merge to form the basilar artery (BA) which supplies the posterior circulation. Branching off the BA to both sides is the posterior cerebral artery (PCA). Many other arteries such as the superior cerebellar, pontine and anterior inferior cerebellar arteries branch off the BA, supplying infratentorial structures such as the cerebellum and brain stem.
The two anteriorly projecting ACAs, are connected by the anterior communicating artery (ACommA). The posterior communicating artery (PCommA) connects the PCA to the ICA, just before its terminal bifurcation into the MCA and ACA. The communicating arteries form the Circle of Willis – a circulatory anastomosis – which supplies blood to the brain. Having a circle of collateral circulation is physiologically significant, for if one part of the circle were to become occluded, blood from collateral vessels of the circle can preserve cerebral perfusion, preventing ischaemia.
See more in the Fundamentals section of aSAH
ANATOMY OF TBI SYMPTOMS
The neural pathways that control pupillary dilatation and constriction are complex.
In the context of trauma, the cause is usually either:
> CN III injury
> Direct ocular trauma
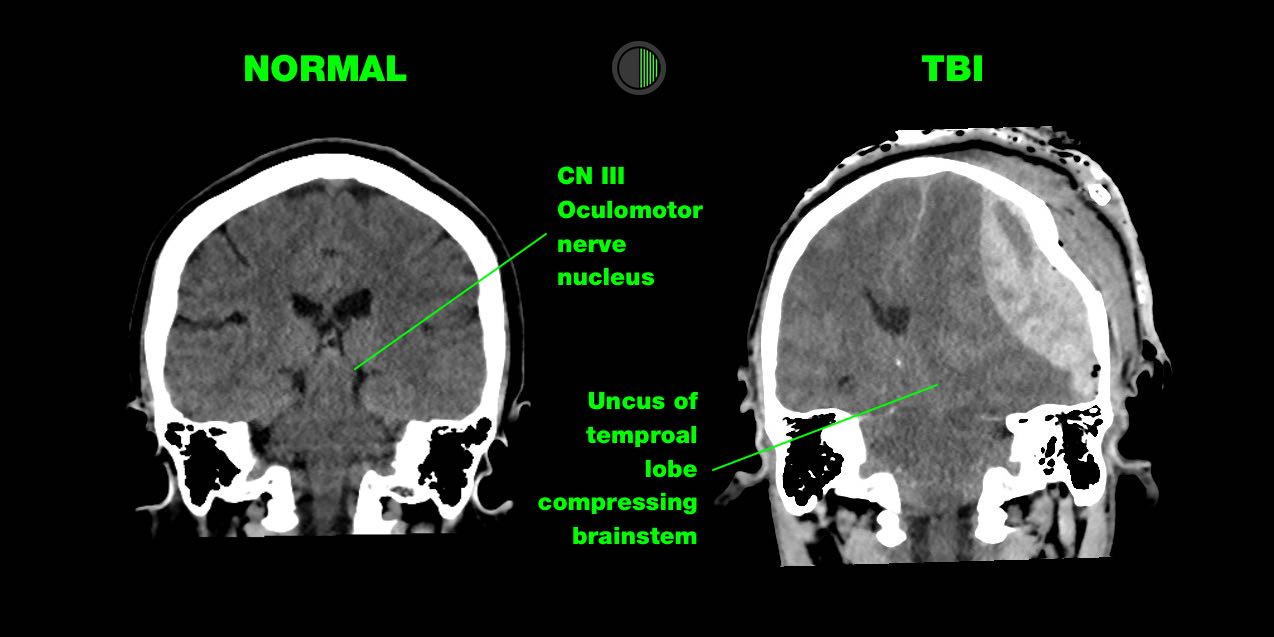
CN III
Injury to CN III (oculomotor) nerve is the most common cause of a unilateral and dilated pupil in trauma.
This happens in 2 ways:
> Direct injury:
CN III is trapped at the tentorial edge as the uncus of the temporal lobe is herniated downward and medially, causing direct injury to CN III
> Ischaemia:
A supratentorial mass causing downward shift of the brain stem, which deforms the perforating brain stem arteries arising from the basilar artery, or global reduction in whole-brain cerebral blood flow that occurs because of raised ICP.
The parasympathetic pupillomotor fibres cause pupil constriction.
They run along the outside of CN III so are more susceptible to direct compression of the nerve.
They also have a separate blood supply (from the pia mater), which is also more sensitive to local and general compression.
This explains why CN III injury in TBI usually only results in a dilated pupil, and not a full CN III palsy, with the eye down and out and a ptosis, which is seen e.g. after surgery for a PCA aneurysm.
Ocular Trauma
> Usually evidence of external trauma around the orbit
Damage to nerve endings in sphincter muscle of iris may result from direct trauma, causing unilateral fixed dilated pupil
More on the pathway involved
WHAT CAUSES STRUCTURAL COMA?
Three types of structural coma:
> Bilateral widespread cortical injury
> Bilateral diencephalon (thalamus, hypothalamus) injury
> Ascending arousal system injury
For more details see the coma fundamentals section
Abnormal posturing response typically to noxious stimuli
> Extension of upper and lower limbs
Mechanism
Classically described as damage to brainstem at or below level of red nucleus
This was deduced when monkeys had cortex removed from this level upwards.
Decerebrate posturing can be seen in patients with large bilateral forebrain lesions with progression caudally into the diencephalon and midbrain.
It can also be caused by posterior fossa lesions compressing the midbrain or rostral pons.
Though decerebrate posturing implies a destructive structural lesion, it can also be caused by reversible metabolic disturbances e.g. hypoglycemia and hepatic encephalopathy
Through animal models and human studies, it has been shown that the vestibulospinal tract plays a major role in decerebrate posturing.
The vestibulospinal pathways have an excitatory effect on extensor motor neurons in the spine, while inhibition of flexor motor neurons.
The vestibular nuclei receive input from the vestibular apparatus and spinal somatosensory pathways while receiving modulatory signals from the cerebral cortex and the fastigial nucleus of the cerebellum.
In isolation, the vestibular nucleus, via the vestibulospinal tract, causes activation of extensor motor neurons in the spinal cord and inhibition of flexor motor neurons.
However, under normal physiology, the higher brain centers of the cortex and cerebellum inhibit the vestibular nuclei, thus preventing this reflex.
Decerebrate posturing results from a disconnection between the modulatory higher centers and the vestibular nuclei, resulting in unsuppressed extensor posturing REF
Abnormal posturing response typically to noxious stimuli
> Flexion of upper limbs and extension of lower limbs
> De-cor-ticate – Arms to the “core”
Mechanism
Classically described as damage to corticospinal tract above level of red nucleus
The rubrospinal tract carries signals from the red nucleus to the spinal motor neurons.
Extensive lesions involving the forebrain, diencephalon, or rostral midbrain are known to cause decorticate posturing. This includes the motor cortex, premotor cortex, corona radiata, internal capsule, and thalamus
In primates, the rubrospinal tract descends as far as the thoracic spine, it, therefore, has effects on the upper limbs but not lower.
The red nucleus, via the rubrospinal tract, causes a flexion, grasping type reflex of the upper limbs.
The higher brain centers, such as the cerebral cortex, inhibit this reflex during normal physiology.
With a lesion of the corticospinal tract, the red nucleus is disinhibited, and the flexion reflex of the upper limbs is unimpeded.
The vestibulospinal tracts, as discussed above, are also left disinhibited, and extension of the lower limbs occurs.
This flexion of the upper limbs and extension of lower limbs is decorticate posturing.
The red nucleus is anatomically at the intercollicular level, and thus lesions above the red nucleus tend to cause decortication and lesions below, decerebration.
As compression advances from the regions of the forebrain and diencephalon to the brainstem, abnormal posturing can progress from decorticate to decerebrate.
Whilst headaches may not be surprising post TBI, they may become a chromic and debilitating consequence of TBI.
Suggested pathogenesis of post-traumatic headaches (PTHA) include:
Impaired Descending Modulation
This theory derives from the similarity between migraine and PTHA.
TBI may result in diffuse axonal injury that may result in structural remodeling of cortical and subcortical regions in the somatosensory and the insular cortex, leading to impaired neuromodulation of descending pain-modulating pathways.
Neurometabolic Changes
TBI changes brain metabolic activity and the physical trauma leads to cellular injury, resulting in an unregulated ion-exchange neurotransmitter release.
The increased neuronal activity results in metabolic stress (lactate and free radicals), leading to axonal damage
Cortical spreading depression is also seen in migraine and is thought to contribute to secondary injury after TBI and, therefore, may play a role in PTHA.
The cellular depolarization in CSD causes an excessive glutamate and potassium release resulting in increased neuronal excitability and activating the trigeminal sensory system.
Trigeminal Sensory System Activation
The trigeminal sensory system may also be affected by neuroinflammation—inflammatory processes in the brain increase after TBI. Neuroinflammation may also increase the central nervous system (CNS) excitability resulting in CSD and subsequent activation of the trigeminal sensory system.
The trigeminal system can also undergo stimulation by nociceptive signals from upper cervical afferents due to overlap in their respective signaling tracts.
The convergence between cervical afferents and trigeminal nerve pathways supports the observation that treatments to cervical neck pain generators can help alleviate PTHA.
Other Theories
Additional possible contributions in PTHA include hyperadrenergic activity, activation of extracranial dural afferents, and meningeal irritation due to craniotomy.
Glymphatic pathway dysfunction also carries implications in PTHA
Do watch this video.
Types:
Previous TopicNext Topic